
We’ve likely all experienced fatigue, either after a long work week or a strenuous training block. Fatigue is typically described as a sensation of lethargy, unable to maintain or increase effort. If you are following our methodology at Tame Pain, then you might notice your session Rate of Perceived Exertion (sRPE) increasing as a reflection of accumulating fatigue. We’ve previously discussed the factors influencing fatigue management by monitoring training load - see HERE. But for this article, let’s take a closer look at the regulators of fatigue beyond just the subjective and objective markers we typically track with training. Rather, let’s discuss what fatigue is and why it occurs.
What is fatigue?
Jones et al define fatigue
“as the decrease in the pre-match/baseline psychological and physiological function of the athlete. An accumulation of fatigue can result in overtraining, which has a significant negative impact on performance.” [2]
We are highly adaptable organisms functioning as a dynamical system in our environment. With respect to exercise, we adapt on a spectrum. Excessive volume or intensity of exercise beyond what someone has adapted to can have detrimental effects. Conversely, with too little exercise an individual may lose the ability to accomplish the daily tasks necessary to remain independent. In other words, there appears to be an optimal range with respect to exercise and performance [3]. But what allows one person to push beyond their prior mental and physical barriers to win a competition, versus another who is presented with the same task demands and is unable to succeed?
Control Mechanisms
We have control processes in place to ensure we meet the demands of the task in an optimal manner, without exceeding homeostatic constraints. Homeostasis will be a reoccurring discussion throughout this piece. Homeostasis, originally discussed by Claude Bernard and later coined by Walter Cannon, is defined as the ability of complex organisms to maintain their internal environment regardless of perturbations from the external world. [4] The purpose of constraint mechanisms is to manage the risk of detrimental effects to the athlete completing the task.
The control processes and their coordinated interactions are a point of debate and research is regularly seeking new perspective on how we are able to accomplish task demands. The fields of biomechanics, psychology, and exercise physiology all seek to explain task completion and exercise performance. Biomechanics discusses motor control and coordinated musculoskeletal action in order to accomplish human movement tasks. The field of psychology discusses task accomplishment based on motivation and goal acquisition. Exercise physiology seeks to understand the interactions between multiple organ systems in order to accomplish a task. Geneticists link specific genes as determinants of physical performance abilities. This list can go on as each person from their respective field seeks to discover the answer to fatigue from their chosen lens.
Single Regulators
There are two prior schools of thought based on “single regulators”: central and peripheral fatigue.
Central fatigue: This premise postulates that the brain is the ultimate regulator of exercise performance, affecting, for example, neurotransmitter signaling or the firing rate of our skeletal muscles. Often, psychological drivers and regulators (motivation) are also included in this category.
Peripheral fatigue essentially describes a supply and demand problem. The “demand” (exercise performance) is too great, and our bodies can’t keep up with the supply (blood, oxygen, ATP, etc.) to our working muscles.
But these single regulator approaches are reductionist and insufficient explanations for the complexities of exercise performance and fatigue beyond central and peripheral regulators. Enter the “Integrative Governor Theory” of fatigue by Gibson et al [1].
Purpose
Rather than separately analyzing each of the individual regulators in task completion and fatigue, Gibson et al put forth a new concept - the Integrative Governor Theory. Integrative Governor Theory defined:
“Integrative Governor theory, which suggests that instead of regulation of activity being either ‘centrally’ or ‘peripherally’ based, or a combination of both, that regulation of activity is created by competition between psychological drives and physiological protection requirements, and that both of these are underpinned by similar basic control mechanisms such as homeostasis and negative feedback loop mechanisms.” [emphasis mine] [1]
The authors propose 8 rules of their theory.
The "8 Rules" of the theory
Rule 1: “...exercise regulation is the result of a pacing/power output choice strategy generated as a result of the competing requirements of physiological protective and psychological success-related drives.” In other words, physiological protective mechanisms are constantly competing with psychological drivers for success (winning), and performance is the product of weighting of those two factors over time (see Fig. 1).
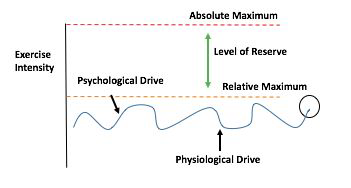
The example provided suggests that athletes who have a “strong physiological protective drive” most likely complete events, but it’s unlikely that the performance was optimal for the individual’s abilities. Conversely, athletes with “too much psychological drive” are likely capable of pushing the limits of their physiology and unable to finish the event or are worse off after the event. Starting and completing an athletic event typically satisfies our psychological drive, but it is also being weighed against the need to reduce exercise intensity based on the degree of discomfort being endured, or even the need to cease activity to protect peripheral physiological systems for survival purposes.
Final thought on rule 1: Power output at any given time represents the product of the relative weighting of the physiological and psychological drivers, which oscillates over the course of an athletic event to determine the pacing profile. Throughout competition, these drivers are dynamically adapting: “increasing as a result of psychological drive requirements, and decreasing as a result of physiological protective mechanisms .” Ultimately the crux of these drivers is the principle of homeostasis, leading to our next two rules.
Rule 2 & 3. Negative Feedback Loops and Set-points
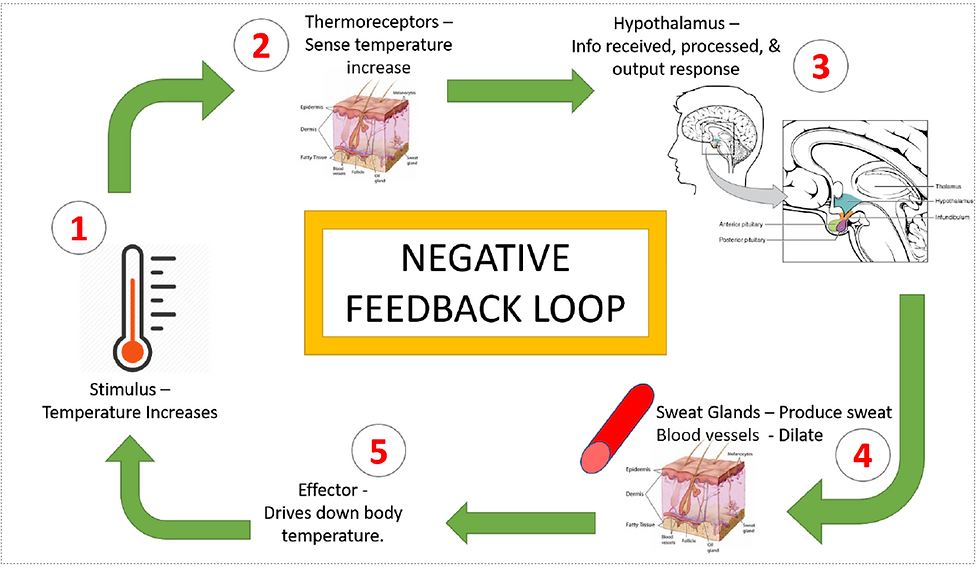
An easy example to understand a negative feedback loop is the hypothalamus as our thermostat. The three fundamental components of negative feedback loops:
Sensory apparatus - detects a change in the system (body) and/or environment.
Control structure - receives information from the sensory apparatus, weighs the information and decides on appropriate response.
Effect mechanism - effects systemic response based on the control structure’s input.
In our example, the thermoreceptors in the skin are our sensory apparatus, the
hypothalamus acts as control structure, and our effect mechanisms are the vascular
system, sweat glands, etc. Thermoreceptors in the skin detect increased body
temperature, this sensory information is sent to the hypothalamus which then decides the body needs to be cooled by sending pertinent information to the sweat glands, vascular system, and other peripheral body tissues (effect mechanism). The sweat glands produce sweat and blood vessels dilate as part of the coordinated response to decrease body temperature.
Negative feedback loops may operate within a single organ system, or in the case of exercise may span multiple systems (brain, cardiovascular, respiratory,
musculoskeletal, etc). Multiple negative feedback loops increase complexity, but also robustness of the system. If systems throughout our brain and body are working in an integrated manner through negative feedback loops, then some “set-point” must exist in order to know when, if, and how much system(s) should alter activity. Rule 3 states, metabolic setpoints are a necessary part for negative feedback loops to function.
As a regulatory system, negative feedback loops compare the current level of activity demands to prior physiological level activity demands in order to return the system to “baseline”. This state of consistent flux in an effort to achieve setpoints can be thought of as allostasis. It’s important to mention, these setpoints aren’t absolute values and more of ranges. One way to think about these metabolic
setpoints is as a level (see figure 3).

The metabolic setpoints are in place as the bookends for allowable ranges without detrimental effects to the athlete. Ideally, the bubble in the level is maintained at an optimal point between these bookends, allowing for successful completion of the athletic event.
Negative feedback loops appear to operate in a Bayesian manner. In other words, prior experiences and expectations are used to set the initial pacing strategy for an exercise activity, but this pace is actively modified as new sensory information comes in over the course of the activity. The authors state,
“Changes in the function or activity of any system is the result of changes occurring external to the system being controlled.” [1]
This leads into our next rule.
Rule 4: Teleoanticipation
Teleoanticipation - “... knowledge of the endpoint of the event is the crucial necessary factor which sets the levels of activity for a particular event or exercise bout. ”
For example, the pacing set by a runner setting up for a 10 km race will be VERY
different than the pace they set for a 100 km race. In this way, external factors (such as the distance of exercise bout) can greatly influence the pacing strategy/physiological activity of the system to meet the task demands. Taking all of this into account, what happens when an athlete is met with varying external factors, perhaps beyond what was expected?
Rule 5. Oscillations to accommodate perturbations
Negative feedback loops act as control mechanisms for psychological and physiological processes in order to maintain physiologic set-points. However, there is an inherent delay in the action of these control mechanisms due to
receiving of sensory information from the surrounding environment,
perturbations to the system,
processing of information, and
enacting appropriate responses.
As a result, our systems are in a continuous state of flux or “oscillation”. The oscillations will vary in amplitude and frequency based on the circumstances, but zero oscillation (i.e., a constant, steady-state ) is likely unobtainable in a dynamic living system. In other words, the only way zero oscillation can occur is likely death.
To further complicate the function of a dynamic system, we have periods of
“uncertainty” while information is being received, processed, and the appropriate
response is being determined. Once sufficient time has elapsed for these steps to
occur, the control mechanisms reach a state of “certainty”, in which responses will be enacted, or the course will be maintained. In essence, there is a constant oscillation between periods of certainty and uncertainty as we take in sensory information and act upon it.
The authors use the effect of blood glucose on insulin and glucagon. Insulin is an
endocrine hormone designed to shuttle glucose from the bloodstream to places such as the liver and muscles, while Glucagon counter-regulates insulin to shuttle glucose from storage facilities into the bloodstream. There are short-duration negative feedback loops where insulin and glucagon are responding to the
changes in glucose, and glucose is changing due to these hormonal changes (see figure 4).
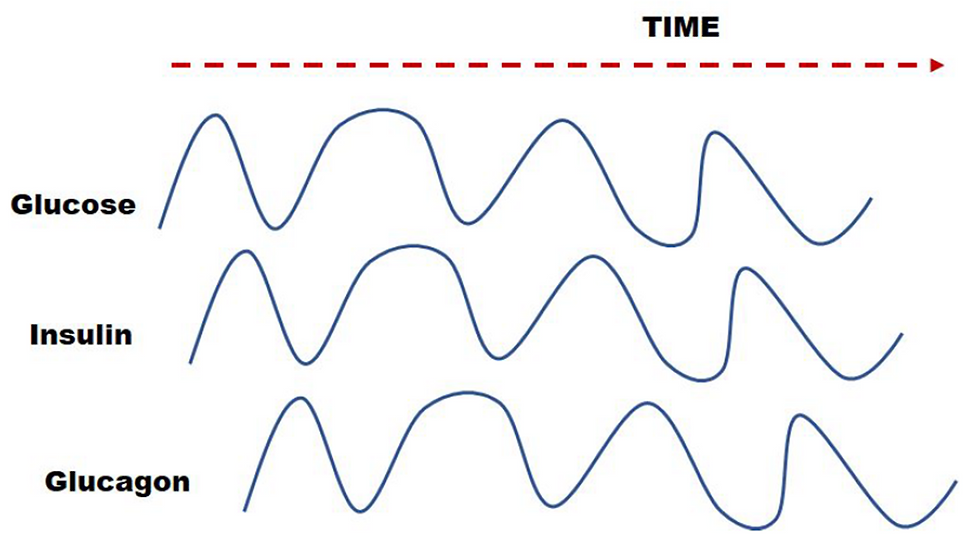
There are also longer-duration negative feedback loops, such as connections between glucose sensors assessing changes in blood glucose concentrations and the brain regulating behavioral changes accordingly. The short- and long-duration loops further increase our complexity and influence our points of certainty and uncertainty. Our next two rules bring everything together.
Rule 6. “The unifying concept underlying all systems is comparison.”
We have to have some prior history, knowledge, or experience upon which we base
future decisions. In the case of exercise, prior activity experiences and current activity demands inform what changes, if any, need to be made to accommodate completion of the task. Interestingly, this need for a base from which to draw from also relates to our subjective rating of difficulty and fatigue levels with measurements like RPE/sRPE and Reps In Reserve (RIR).
Rule 7. Information processing.
According to the authors,
“All changes in any variable in a system generate information, which thus becomes the ‘language’ of communication which operates across different types of systems.” [1]
This ‘language’ is necessary for inter/intra system(s) communication to allow for our overall functioning and performance abilities. The communication process is outlined in Figure 5.

Green box - receive and decipher information.
Orange box - stores information to draw from for current or future decision making, memory.
Response occurs leading to generation of new information (yellow box), leading to awareness of new information that needs to be received and deciphered (negative feedback loop).
Of note, this is a continuous and endless process. With an understanding of the need for comparison and communication, we have our last rule.
Rule 8. Awareness
Why does awareness of system(s) occur? The authors argue because of unexpected changes in the system, another system acting on that system, or discrepancies which occur between strategy and tactics utilized by the governor of the system. Returning to the discussion of fatigue - it has been previously argued that we become consciously aware of fatigue (as a sensation) due to changes in “subconscious homeostatic control systems”. Fatigue arises from, “... a temporal difference between subconscious representations of these homeostatic control mechanisms in neural networks that are induced by changes in the level of activity.”
The perception of effort, therefore, “acts as such a signal in response to increases in exercise intensity that are perceived to be sustainable by the complex, dynamic
subconscious teleological pacing control mechanisms.”
Effort is therefore a separate and distinct sensation in and of itself from any other
physical sensations. The authors go on to argue, “... the sense of effort acts as an intermittent inhibitory sensation, which would compete with psychological drives to continue exercise, the outcome being either:
a willful disregard for the inhibitory sense of effort;
a conscious reduction of exercise intensity to correct the pacing strategy to a potentially successful plan; or
in extreme cases, the cessation of the exercise altogether.”
Why does this matter?
As we can see, the topic of fatigue and regulation of exercise activity is not as
straightforward as previously thought. The prior belief that peripheral or central
mechanisms or a combination of both ruled fatigue is insufficient to explain the complete picture. The Integrative Governor Theory seeks to explain the complexities of the topic by elucidating the “competition between psychological and physiological homeostatic drives”. The theory postulates these drivers are antagonistic to one another, but are regulated by the same mechanisms. The authors ultimately argue,
“... the relative ‘weighting of each’ by a decision-making function or structure in the brain, is what regulates exercise activity, the fatigue process, and the outcome of a particular athletic event for the individual competing in it.” [1]
The crux of these drivers is the principle of homeostasis and a continuous state of
oscillatory activity seeking to find balance of a dynamical system. These oscillations are executed in a Bayesian fashion, drawing from prior experiences and forecasting the future demands of an activity. We have metabolic set-point ranges, which aid negative feedback loops to ensure when and if appropriate alterations are necessary to a particular variable, system, or the whole. We have a universal language via information processing which allows communication intra/inter system(s) ultimately leading to universal control. Finally, it’s important to remember that due to delay of receiving, processing, and determining appropriate reactions (if any) that we oscillate between continuous states of certainty and uncertainty, giving rise to a constant state of flux. The only achievable permanent setpoint with zero oscillations would likely be death.
In closing, we are a dynamical system capable of adapting to varying stressors in order to maintain homeostasis and complete athletic events. Our adaptability allows for improvement in athletic endeavors but why one individual revels in success and another falls just short is multifactorial and difficult to conclusively state a single factor as paramount.
P.S. - It's completely normal to notice your fatigue levels undulating session to session for innumerable reasons beyond dosage of activity. For example, sleep, nutrition, and stress will influence an individual's fatigue levels.
References
St Clair Gibson A, Swart J, Tucker R. The interaction of psychological and physiological homeostatic drives and role of general control principles in the regulation of physiological systems, exercise and the fatigue process - The Integrative Governor theory. European journal of sport science. 2018; 18(1):25-36.
Jones CM, Griffiths PC, Mellalieu SD. Training Load and Fatigue Marker Associations with Injury and Illness: A Systematic Review of Longitudinal Studies. Sports medicine (Auckland, N.Z.). 2017; 47(5):943-974.
Gabbett TJ. The training—injury prevention paradox: should athletes be training smarter harder? Br J Sports Med. 2016; 50(5):273-280.
Modell H, Cliff W, Michael J, McFarland J, Wenderoth MP, Wright A. A physiologist's view of homeostasis. Advances in physiology education. 2015; 39(4):259-66.
Ulmer HV. Concept of an extracellular regulation of muscular metabolic rate during heavy exercise in humans by psychophysiological feedback. Experientia. 1996; 52(5):416-20.
Comments